Basics of Signal Transduction 
- Process by which a chemical or physical signal is transmitted through a cell that includes series of molecular interactions and events, ultimately resulting in a cellular response to the signal
- Messenger attaches to the binding site → shape of the protein changes (allosteric modulation) → cell response
- Membranes, Proteins, and Channels
- Organelle function review
- Cell membrane- receives and transmits signal
- Nucleus: DNA and RNA production
- Ribosomes: protein synthesis
- Endoplasmic Reticulum
- Rough ER: packaging proteins
- Smooth ER: stores lipids and Ca2+
- Golgi Apparatus: post-translational modification
- Endosomes: sorting, trafficking
- Mitochondria: cell respiration
- Cytoskeleton: transport and motility
- Polar molecules have positive and negative ends
- Surround each other with polar molecules
- Water is polar, but lipids are nonpolar and that is why water and lipids do not mix
- Phospholipids have a polar region on one end and nonpolar tails
- Amphipathic molecules
- Spontaneously aggregate to form a membrane with the hydrophobic nonpolar ends in the middle and hydrophilic polar ends on the outside
- Fluid Mosaic Model
- Membrane fluidity: Since there are no chemical bonds between the fatty acid chains, a lot of lateral movement within the membrane is possible
- General structure of the membrane
- Functions of the plasma membrane
- They act as a selective barrier; they regulate the passage of substances into and out of the cell.
- They play a role in communication between cells: they detect chemical signals from other cells.
- They link adjacent cells together by membrane junctions.
- They anchor cells to the extracellular matrix.
- Most of these functions are carried out by membrane proteins
- Integral membrane proteins: closely associated with the membrane lipids and are amphipathic proteins
- Some span the entire membrane and are called transmembrane proteins
- Peripheral membrane proteins: located at the inner or outer membrane surface; do not interact with phospholipid tails
- Inner - Influence cell shape and motility
- Outer - interact with or contain carbohydrate chains

- How do cells connect? Cell Junctions

- Tight junctions: block the flow of fluids between epithelial cells
- Desmosomes: form links between cells, and provide a connection between intermediate filaments of the cell cytoskeletons of adjacent structures - gives strength to tissues
- Gap junctions: form pores connecting adjacent cells - small molecules and electrical signals in one cell can pass through the gap junctions to adjacent cells
- Newly synthesized polar membrane proteins get to the surface/outside the cell in vesicles packaged in the Golgi
- Interactions between proteins and ligands - binding
- A ligand is any molecule bound to a protein
- In biological systems, ligand binding is typically reversible
- Proteins can have many binding sites
- Binding of a ligand to a protein will often change the conformation of the protein
- Affinity: strength of logan-protein binding
- Chemical specificity: selectivity for one or more ligands
- Covalent modulation: covalent bonding of a charged chemical group by an enzyme to a protein that produces a conformational change to the shape of the protein
- Allosteric modulation: a protein contains 2 or more binding sites and the noncovalent binding of a ligand to one site can alter the shape and the characteristics (usually the affinity for a ligand) of the other site
- When an original cell messenger (first messenger) response is relayed by another chemical messenger inside the cell called a second messenger
- Receptors show features of ligand protein binding
- Ligand specificity
- The first messenger binding site has a particular shape into which only certain molecules fit.
- As a result, only certain messengers will elicit a response.
- If one molecule elicits a strong response, structurally related molecules may elicit weaker responses.
- Unrelated molecules usually elicit no response at that receptor.
- Ex. Some adrenergic receptors bind NE > Epi >>>> ACh
- Saturation

- The degree to which the receptors on a cell are occupied by a messenger
- The more receptors are occupied by a messenger, the stronger the cellular response
- If 100% of the receptors are occupied - fully saturated
- Creates an upper limit to the responsiveness of the receptor system
- Affinity vs. Saturation
- \
- Drugs and Receptors
- Agonist: A chemical messenger that binds to a receptor and triggers the normal response.
- The term is often used for a drug that mimics the action of the normal messenger (decongestant drugs phenylephrine and pseudoephedrine mimic the action of epinephrine.)
- Antagonist: A molecule that binds to a receptor but does not elicit a response.
- Some common antagonists are called “blockers”
- Competition
- Different molecules with similar structures will compete for combination with the receptor.
- Certain therapeutic drugs make use of this property.
- E.g. b-blockers are antagonists to epinephrine and norepinephrine for b-receptors.
- Administering b-blockers to a patient will reduce their heart rate and the strength of their cardiac contraction because some of the receptors are occupied by the b-blockers (which do not elicit a response)
- Acclimation: number of receptors in the cell membrane can fluctuate
- Down-regulation: when the number of receptors decrease due to a high extracellular concentration of a messenger for some time
- Result: frequent or intense stimulation by a messenger will reduce the responsiveness of the target cells to that messenger. (Local negative feedback)
- Up-regulation: when the number of receptors increase due to a low extracellular concentration of a messenger for some time
- Result: cells develop increased sensitivity
Membrane Transport
- Diffusion
- The concentration in 1 will be equal to the concentration in 2 and the two fluxes will be the same
- System at diffusion equilibrium - net flux = zero
- Flux: amount of material crossing a surface unit (cm2) per time unit (s)
- The net flux is always from a region of higher concentration to a region of lower concentration
- Substances are said to move downhill
- Diffusion is driven by the concentration gradient
- Larger the concentration gradient, larger the net flux
- The body does not need to supply energy for diffusion
- For a given concentration gradient, the net flux is affected by several factors
- The higher the temperature, the greater the speed of molecular movement and the greater the net flux
- The larger the molecular mass, the lower the speed of the molecules and the lower the net flux
- The larger the available surface area the larger the net flux
- The medium also plays a role: molecules diffuse more rapidly in air than in water
- Diffusion across membranes
- Nonpolar substances
- Since the membrane is made of phospholipids, substances that dissolve in lipid diffuse across the cell membrane
- Ex. oxygen, carbon dioxide, fatty acids, steroid hormones, anesthetics, drugs
- Ions: charged, so they cannot diffuse across the lipid membrane; have special channels created for them
- Channels are small and often selective
- Sodium, potassium, calcium channels
- Partly selective or nonselective
- Channels can either be open or closed; channel gating modifies the conformation of transmembrane proteins
- Gradients
- The concentration gradient drives the diffusion of ions.
- If the Na+ concentration outside the cell is higher than that inside the cell and there are Na+ channels in the membrane that are open, Na+ will diffuse into the cell.
- But, remember that every cell is also like a small battery; the inside of the cell is slightly negative with respect to the outside.
- This will also affect the direction and amount of ion movement.
- Role of electrical forces on ion movement
- Opposite charges attract each other and like charges repel each other.
- Since Na+ ions are positive and the inside of the resting cell is negative, Na+ is attracted to the inside of the cell.
- So, ion movement across membranes is actually governed by an electrochemical gradient.
- Osmosis
- When you have a membrane that is permeable to water but not to solute, water is drawn to the side with the higher solute concentration.
- This is due to the diffusion of water down its concentration gradient, which produces a change in the volume of the compartments.
- In kidneys, both in the proximal convoluted tubule and the loop of Henle, osmosis of water occurs whereby the water moves from the more concentrated environment within the tubules to the less concentrated environment of the capillaries.

- Mediated and vesicular transport systems
- Large, fat-insoluble molecules such as proteins, amino acids and glucose cannot get in or out of the cell by these means. Also, ions can’t move freely through open channels if they have to go “uphill” against their electrochemical gradient
- Both types use a transporter/carrier that is a transmembrane protein that undergoes conformational changes
- Mediated transport requires binding to a transporter, in contrast to simple diffusion which
- occurs either through the membrane lipids or through open channels.
- Transporters follow the basic rules of protein/ligand binding:
- They exhibit specificity.
- They require a conformational change to transport the ligand.
- They are limited in number on the membrane.
- Facilitated diffusion
- Requires a carrier
- Moves downhill
- Large, polar molecules are too large to diffuse through ion channels
- It does not require cellular energy
- It uses a transmembrane protein as a carrier
- It has chemical specificity
- It displays saturation (This happens when all the available carriers are used.)
- Ex. Type 1 Diabetes
- Not all glucose transporters are the same. There are different transporter subtypes in different cells. The ones in muscle and adipose tissue are regulated by the hormone insulin.
- When insulin is present, the number of transporters in the membrane is increased and as a result the flux of glucose into the cell is increased.
- People with type-1 diabetes lack insulin. As a result, glucose cannot enter cells normally. So, glucose levels increase in the blood.
- Active transport
- Active transport moves substances uphill, against their electrochemical gradient
- Requires energy
- Uses a transmembrane protein as a carrier
- Chemically specific
- Displays saturation
- Primary active transport
- Hydrolysis of ATP by the carrier provides the energy
- Transporter itself is an enzyme
- ATPase the catalyzes the breakdown of ATP to ADP
- Secondary active transport
- The transporter protein has two binding sites, one for the solute that needs to be transported uphill and one for the ion (Na+) that provides the energy.
- In secondary active transport, the energy comes indirectly from ATP.
- Thus, the ion that provides the energy is Na+. It moves downhill into the cell
- The other solute is moved uphill
- If the other solute is also moved in the same direction as Na+, we call it cotransport or symport.
- If the other solute is moved out of the cell, we call this: countertransport or antiport.
- Vesicular transport
- Sometimes, large materials must leave or enter the cell. E.g. large protein hormones are secreted by cells, or bacteria are ingested by white blood cells. These materials do not pass through the plasma membrane
- Endocytosis: the plasma membrane folds into the cell and makes a small pocket that encloses the material and eventually pinches off to form a vesicle.
- Exocytosis: vesicles in the cytoplasm fuse with the plasma membrane and release their contents outside of the cell.
- Fluid endocytosis: the endocytotic vesicle simply encloses a small volume of ECF. This is often referred to as pinocytosis (cell drinking)
- Phagocytosis (cell eating): large particles, such as bacteria and debris from damaged tissues, are engulfed by the plasma membrane
- While most cells undergo pinocytosis, only a few specialized cells carry out phagocytosis. The phagocytosis of bacteria and their destruction by the lysosomal digestive enzymes is one of the body’s major defense mechanisms against microorganisms

- Epithelial transport
- Epithelial cells are polarized, meaning they have two membrane surfaces with different permeability characteristics
- They have tight junctions between them so solutes have to go through the cell
- The apical or luminal membrane faces the lumen
- The basolateral or basal membrane faces the blood vessels
- If Na+/K+ pumps are on the basal membrane, this moves Na+ out of the cell into the blood by active transport and Na+ from the lumen into the cell by facilitated diffusion.
- Drugs targeting transporters
- Ezetimibe- Inhibits absorption of cholesterol in the small intestine via the sterol
- transporter, Niemann-Pick C1-Like1 (NPC1L1)
- Uses: Primary hyperlipidemia, Homozygous familial, hypercholesterolemia
- Canagliflozin, dapagliflozin, and empagliflozin are approved as SGLT2 (Sodium glucose co-transporter) inhibitors for clinical use in diabetes mellitus, type 2
Diuretics Furosemide and Hydrochlorothiazide- Inhibits the activity of the Na+-K+-2Cl− symporter in kidneys and enhance the excretion of water along with electrolytes
Signal Transduction Pathways
Kinase: enzyme that adds phosphate groups
Phosphatase: enzyme that removes phosphate groups
Tyrosine Kinases
- Receptor Tyrosine Kinases (RTK): receptors with intrinsic tyrosine kinase activity
- Non-receptor Tyrosine Kinase (NRTK): intracellular proteins that are responsible to phosphorylating a variety of intracellular proteins on tyrosine residues
- NRTKs may also be referred to as protein tyrosine kinases (PTKs)
Protein phosphorylation
- In eukaryotic cells: serine, threonine, and tyrosine are amino acids with nucleophilic hydroxyl groups
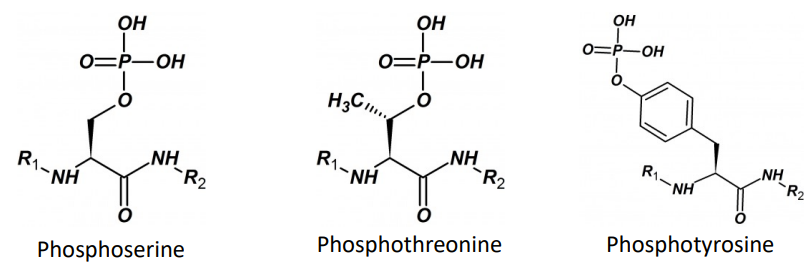
- Protein phosphorylation is a reversible reaction that is mediated by kinases and phosphatases, which phosphorylate and dephosphorylate substrates, respectively
RTKs
- Ligand-regulated transmembrane enzyme
- 59 members of human genome
- Most RTKs are monomers and their domain structure includes
- An extracellular ligand-binding domain
- A transmembrane domain
- An intracellular domain possessing the tyrosine kinase activity
- RTKs have a wide range of ligands
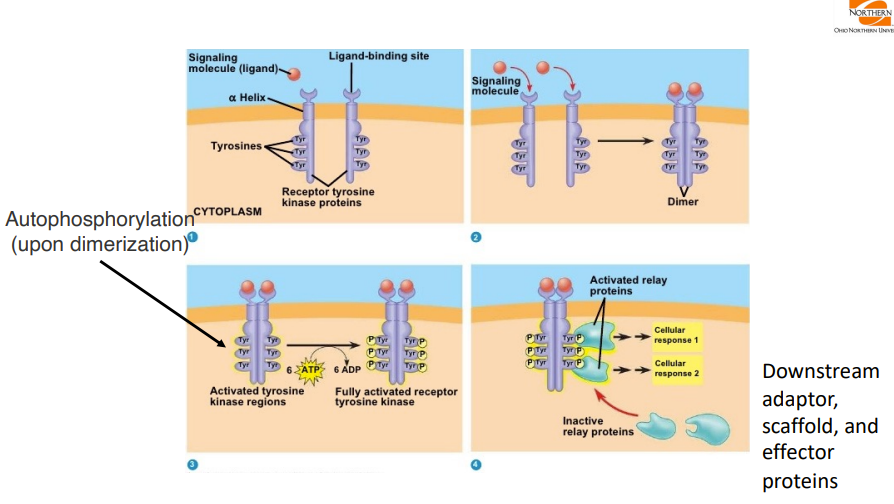
- insulin, epidermal growth factor (EGF), platelet-derived growth factor (PDGF), atrial natriuretic peptide (ANP), transforming growth factor-β (TGF-β), and many other trophic hormones.
- STEP 1: Receptor tyrosine kinases start out as inactive monomers. Note that each ligand has a binding site.
- STEP 2: Upon binding, the receptor forms a dimer of proteins that phosphorylate each other
- Step 3: The activated tyrosine
Phosphorylated RTKs
- The phosphorylation of other tyrosine residues forms docking sites for several proteins, including SH2 domains non-receptor tyrosine kinases
- Examples of SH2 domain-containing proteins
- PLCγ: the activity of which raises intracellular levels of Ca2+ and activates PKC
- PI3K: increase the level of PIP3 and PKB (also known as Akt)
- Grb2: adaptor molecule without activity
- Mitogen-activated protein kinases
- Three main groups
- Extracellular signal-regulated kinases (ERK)
- The p38 family
- JUN amino-terminal kinases (JNK)
NRTKs and RTKs are a common drug target for oncology
RTK Inhibitors
- Erlotinib is a potent inhibitor of the EGFR tyrosine kinase
- Competitively inhibits ATP binding at the active site of the kinase
- Non-small cell lung cancer, metastatic
- Pancreatic cancer
- Cetuximab is a monoclonal antibody to the extracellular domain of the EGFR
- Binds specifically to the extracellular domain of EGFR and prevents ligand-dependent signaling and receptor dimerization
- Colorectal cancer, metastatic
- Head and neck cancer, squamous cell
Cytokine receptors
- Respond to a heterogenous group of peptide ligands, which include growth hormone, interleukins, erythropoietin, several kinds of interferons, and other regulators of growth and differentiation
- Mechanism resembles RTK
- Activation
- Protein tyrosine kinase, from the Janus-kinase (JAK) family, binds noncovalently to the receptor
- Cytokine receptors dimerize after they bind the activating ligand
- JAKs become activated and phosphorylate tyrosine residues on the receptor
- Phosphorylated tyrosine residues bind to another set of proteins, called STATs (signal transducers and activators of transcription).
- Bound STATs are themselves phosphorylated by the JAKs
- Two STAT molecules dimerize (attaching to one another’s tyrosine phosphates)
- Finally, the STAT/STAT dimer dissociates from the receptor and travels to the nucleus, where it regulates transcription of specific genes.
- Type I cytokine receptors
- Presence of two pairs of conserved cysteines linked via disulfide bonds and fibronectin type II modules in the extracellular domain
- Type II cytokine receptors
- Also have two pairs of conserved cysteines but with a different arrangement to Type I and also lack the WSXWS motif
Nuclear receptors
- Comprise a superfamily of 48 receptors that respond to a diverse set of ligands
- Situated inside the cell either in cytoplasm or nucleus
- Several biologic ligands are sufficiently lipid-soluble to cross the plasma membrane and act on intracellular receptors
- Type I: Steroid sex hormones receptors for androgen, estrogen, and
- progesterone, glucocorticoid and mineralocorticoid receptors
- Type II: Thyroid hormone, vitamin A & D and retinoid receptors
- Type III and IV: not prominent/discussed
- Structure
- A highly variable N-terminal domain that includes several distinct transactivation regions (AF-1)
- A central conserved DNA-binding domain (two zinc fingers)
- C-terminal hinge region which forms ligand-binding domain
- Amino acid residues for binding coactivators and corepressors in a second activation region (AF-2)
- Can exist as monomers, homodimers, or heterodimers
- Recognize DNA sequences termed hormone response elements (HREs) and stimulate the transcription of genes

Ion channels
- Flux of ions across the plasma membrane is a critical regulatory event in both excitable and non-excitable cells, but the lipid bilayer is impermeable to anions and cations
- To maintain membrane potential, all cells have ion transporters for Na+, K+, Ca 2+, Cl-
- Passive ion fluxes down cellular electrochemical gradients are regulated by a large family of ion channels located in the membrane
- Humans express ~232 distinct ion channels to precisely regulate the flow of ions across the membrane
- Voltage-gated ion channels
- Conduct ions at high rates
- Regulated by the voltage across the membrane
- Ex. VG sodium channel is involved in the generation of action potential in nerves and cardiac cells
- Sodium channel blockers are effective against partial seizures; ex. Carbamazepine, oxcarbazepine, lamotrigine, phenytoin, topiramate
- Ex. VG calcium channel regulates entry of calcium inside cardiac and smooth muscle cells
- Blocking CC’s leads to vasodilation; can treat angina, cardiac arrhythmias, HTN (nifedipine, diltiazem, verapamil)
Ligand-gated (LG) ion channels
- Ion channels are activated by the binding of a ligand to a specific site on the channel protein (ionotropic receptors)
- Major LG ion channels in the nervous system are those that respond to:
- Excitatory neurotransmitters such as ACh or glutamate
- Inhibitory neurotransmitters such as glycine or γ-aminobutyric acid (GABA)
- Ex. Benzodiazepines act at GABAA receptors by binding directly to a specific site that is distinct from that of GABA binding.
- The benzodiazepines do not substitute for GABA but appear to enhance GABA’s effects allosterically without directly activating GABAA receptors or opening the associated chloride channels.
- Pharmacological effects include: anxiolytic, sedation, hypnosis, anesthesia, muscle relaxation
\n
Signal Transduction Pathways (GPCRs)
- Properties
- GPCRs regulate a vast number of physiological functions including:
- Nerve activity
- Tension of smooth muscle
- Metabolism
- Rate and force of cardiac contraction
- Secretion of most glands in the body
- The ligands for GPCRs include:
- Neurotransmitters such as acetylcholine (ACh),
- Biogenic amines such as Norepinephrine, all eicosanoids and other lipid signaling molecules,
- Peptide hormones
- Amino acids such as GABA, and many other peptide and protein ligands
- Classification

- GPCRs are classified into six classes based on sequence homology and functional similarities:
- Class A - Rhodopsin-like receptors
- Class B - Secretin receptor family
- Class C - Metabotropic glutamate receptors
- Class D - Fungal mating pheromone receptors
- Class E - Cyclic AMP receptors
- Class F - Frizzled and Smoothened receptors
- G Proteins have 3 subunits
- Gα – the guanine nucleotide-binding subunit; has innate GTPase activity which acts to terminate the signal
- Gβ and Gγ subunits are tightly bound together that they do not dissociate and are therefore written as Gβγ
- Gα: several types of Gα protein exist and these produce distinct effects
- Gαs (stimulating) subunit stimulates adenylyl cyclase activity (cAMP)
- Gαi (inhibitory) subunit inhibits adenylyl cyclase activity (cAMP)
- Gαq activates phospholipase C (Ca2+)
- Gα12/13 couple to guanine nucleotide exchange factors (GEFs)
- Adrenergic receptors (sympathetic nervous system)
- Alpha-1 receptors: Gq
- Alpha-2 receptors: Gi
- Beta-1 receptors: Gs
- Beta-2 receptors: Gs
- Beta-3 receptors: Gs
- Muscarinic acetylcholine receptors (parasympathetic nervous system)
- M1, M3, M5 receptors: Gq
- M2 and M4 receptors: Gi
- Dopamine receptors
- D1-like receptors (D1 and D5):Gs
- • D2-like receptors (D2, D3, and D4): Gi
- Histamine receptors
- Remember with GCPRs…
- Receptor the drug is acting on
- Which type of G-protein that is associated with the receptors
- What the downstream effect of the protein is
- GPCR Mechanism of Action
- Not a channel or enzyme; receptor is linked through G-protein to effector proteins such as channels or enzymes
- When the first messenger attaches to the receptor, the change in shape of the receptor causes part of the G-protein to dissociate and diffuse along the inner surface of the plasma membrane to link up with an effector protein (which can be an ion channel or an enzyme).

- These ion channels or enzymes then mediate the next steps in the sequences of events leading to the cell’s response.
- The G-protein is an additional link in the chain. It can amplify the response.
- If the effector protein is a channel, the G-protein may cause the channel to open or close

- If the effector protein is an enzyme, the cell will generate a second messenger, which will serve as a relay from the plasma membrane to the biochemical machinery inside the cell
- The more first messenger that attaches to the receptor, the more second messenger is produced and the stronger the cellular response
- Receptor Regulation - what if there is a constant presence of ligand/agonist?
- After reaching an initial high level, the response (eg, cellular cAMP accumulation) diminishes over seconds or minutes, even in the continued presence of the agonist
- Known as receptor desensitization
- This “desensitization” is often rapidly reversible
- A second exposure to agonist, if provided a few minutes after termination of the first exposure, results in a response similar to the initial response.
- Desensitization
- The agonist-induced change in conformation of the receptor causes it to bind, activate, and serve as a substrate for a family of specific receptor kinases, called G protein-coupled receptor kinases (GRKs).
- The activated GRK then phosphorylates serine residues in the receptor’s carboxyl terminal tail.

- The presence of phospho-serine increases the receptor’s affinity for binding a third protein, β-arrestin.
- Binding of β-arrestin to cytoplasmic loops of the receptor diminishes the receptor’s ability to interact with Gs, thereby reducing the agonist response (i.e., stimulation of adenylyl cyclase).
- Upon removal of agonist, GRK activation is terminated, and the desensitization process can be reversed by cellular phosphatases.
- Homologous desensitization: Specific G protein-coupled receptor kinases (GRKs) selectively phosphorylate agonist-activated receptors
- Inactivate the agonist-activated receptor
- Heterologous desensitization: Second messenger-dependent protein kinases not only phosphorylate agonist-activated GPCRs, but also indiscriminately phosphorylate receptors that have not been exposed to agonist

- Secondary messenger: cGMP
- cGMP has established signaling roles in only a few cell types
- Example: Intestinal mucosa and vascular smooth muscle
- cGMP is synthesized by receptor guanylyl cyclases and soluble cytoplasmic guanylyl cyclases
- Atrial natriuretic peptide, a blood-borne peptide hormone, activates the receptor guanylyl cyclases
- Nitric oxide is a known activator of soluble cytoplasmic guanylyl cyclases
- cGMP leads to the activation of the cGMP-dependent protein kinase (PKG)
- PKG phosphorylates some of the same substrates as PKA and some that are PKGspecific
- Important effects of elevated cGMP include modulation of platelet activation and relaxation of smooth muscle
- Phosphoinositides and Calcium
- Calcium is an important messenger in all cells and can regulate diverse responses including:
- Gene expression
- Contraction
- Secretion
- Metabolism
- Electrical activity
- Ca2+ can enter the cell through Ca2+ ion channels in the plasma membrane or be released from intracellular stores
- GPCRs that couple to Gq activate phospholipase c β (PLCβ) by activating the G protein α subunit and releasing the βγ dimer
- PLCs are cytosolic enzymes that translocate to the plasma membrane upon receptor stimulation.
- The calcium-phosphoinositide and cAMP signaling pathways oppose one another in some cells and are complementary in others
- Smooth muscles: opposing effects
- IP3-mediated mobilization of Ca2+ causes contraction
- Elevation of cAMP causes relaxation
- Liver: complementary phenomenon
- cAMP and phosphoinositide second messengers act together to stimulate glucose release
- Biased Agonism
- GPCR-ligands are classified based on their efficacies for activation of G-proteins
- Full agonists, partial agonists, antagonists, or inverse agonists - depending on their abilities to elicit a receptor-mediated response
- β-arrestins, which have been long associated with receptor desensitization, can also lead to signaling events
- It is now evident that β-arrestins regulate GPCR trafficking as well as G-protein-independent signaling
- Ex. Carvedilol - antagonist for beta-1 and beta-2 adrenergic receptors, so the drug is going to block both these receptor subtypes on cardiac tissue